Rarest nucleus reluctant to decay
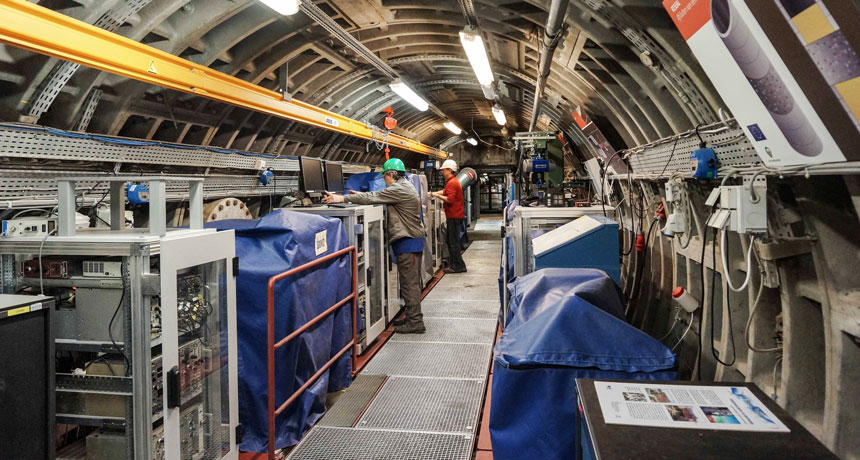
Nature’s rarest type of atomic nucleus is not giving up its secrets easily.
Scientists looking for the decay of an unusual form of the element tantalum, known as tantalum-180m, have come up empty-handed. Tantalum-180m’s hesitance to decay indicates that it has a half-life of at least 45 million billion years, Bjoern Lehnert and colleagues report online September 13 at arXiv.org. “The half-life is longer than a million times the age of the universe,” says Lehnert, a nuclear physicist at Carleton University in Ottawa. (Scientists estimate the universe’s age at 13.8 billion years.)
Making up less than two ten-thousandths of a percent of the mass of the Earth’s crust, the metal tantalum is uncommon. And tantalum-180m is even harder to find. Only 0.01 percent of tantalum is found in this state, making it the rarest known long-lived nuclide, or variety of atom.
Tantalum-180m is a bit of an oddball. It is what’s known as an isomer — its nucleus exists in an “excited,” or high-energy, configuration. Normally, an excited nucleus would quickly drop to a lower energy state, emitting a photon — a particle of light — in the process. But tantalum-180m is “metastable” (hence the “m” in its name), meaning that it gets stuck in its high-energy state.
Tantalum-180m is thought to decay by emitting or capturing an electron, morphing into another element — either tungsten or hafnium — in the process. But this decay has never been observed. Other unusual nuclides, such as those that decay by emitting two electrons simultaneously, can have even longer half-lives than tantalum-180m. But tantalum-180m is unique — it is the longest-lived isomer found in nature.
“It’s a very interesting nucleus,” says nuclear physicist Eric Norman of the University of California, Berkeley, who was not involved with the study. Scientists don’t have a good understanding of such unusual decays, and a measurement of the half-life would help scientists pin down the details of the process and the nucleus’ structure.
Lehnert and colleagues observed a sample of tantalum with a detector designed to catch photons emitted in the decay process. After running the experiment for 176 days, and adding in data from previous incarnations of the experiment, the team saw no evidence of decay. The half-life couldn’t be shorter than 45 million billion years, the scientists determined, or they would have seen some hint of the process. “They did a state-of-the-art measurement,” says Norman. “It’s a very difficult thing to see.”
The presence of tantalum-180m in nature is itself a bit of a mystery, too. The element-forging processes that occur in stars and supernovas seem to bypass the nuclide. “People don’t really understand how it is created at all,” says Lehnert.
Tantalum-180m is interesting as a potential energy source, says Norman, although “it’s kind of a crazy idea.” If scientists could find a way to tap the energy stored in the excited nucleus by causing it to decay, it might be useful for applications like nuclear lasers, he says.